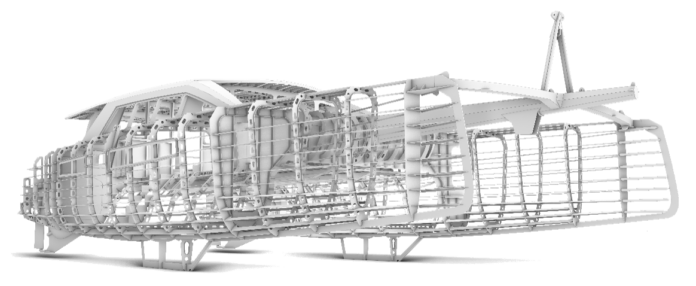
Even a quick glance at the commercial maritime industry highlights the value of aluminum vessels. Their inherent durability, strength and resistance to corrosion makes them ideal for commercial applications since they avoid excessive wear and maintenance. Aluminum is a low-density material, which allows for reasonably low structural weights, making it a good fit for applications where time is of the essence. Flat panel boats with hard chines and developable cabins, are easily constructed and can be cost-effective. Considering this, it’s no surprise to see the growing interest and demand for aluminum cruisers in the market, especially when buyers want a robust vessel.
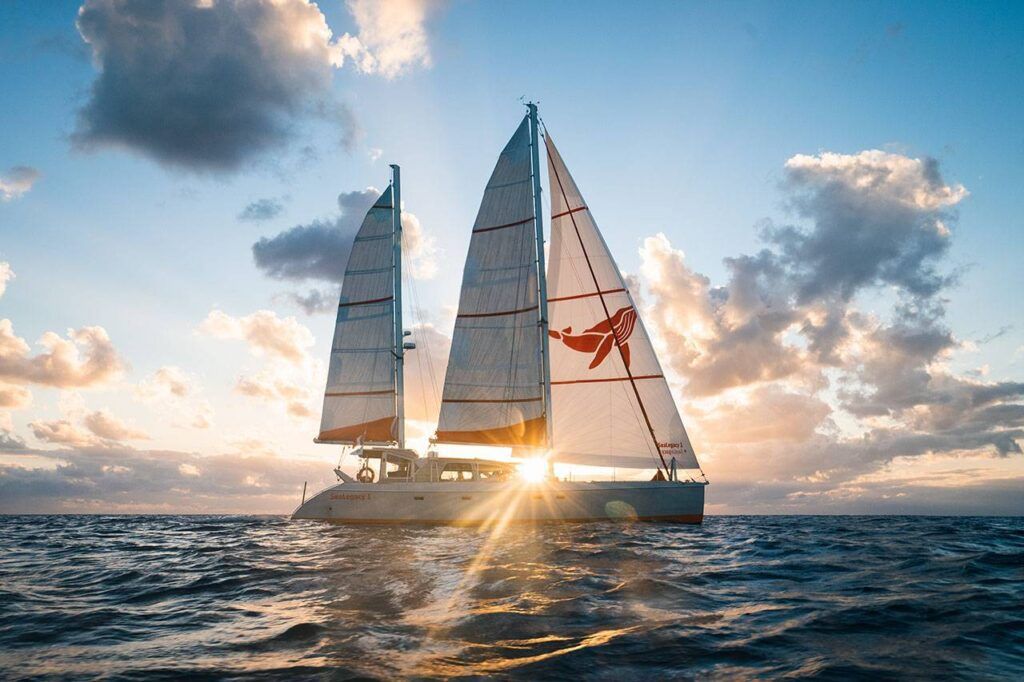
When it comes to cruisers, rather than competing against steel commercial boats, aluminum’s largest contender is fiberglass—the material of choice for production boats. Churned out of large factories optimized to reduce build cost, they’re a good option for well-presented boats with decent performance while not breaking the bank. However, as most practical sailors know, there are many things that can, and do, go wrong. It’s not hard to find examples of osmosis, delamination and core failures in well-used boats, but structural failures are now being found in near-new production boats as well. Higher quality custom composite boats won’t share these issues, but that certainly comes at a price as the efficiencies of scale are lost when only building a few of a design.
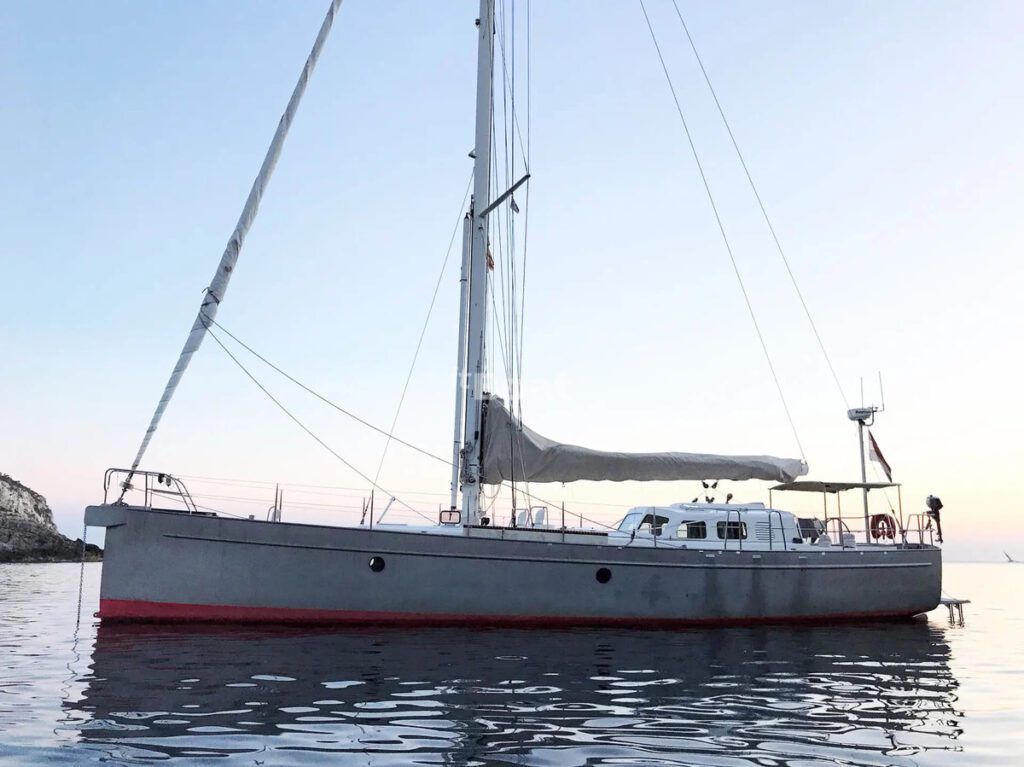
This gap in the custom market has presented an opportunity for aluminum designs, which can be built cost-effectively in small numbers from CNC cut build kits. For an owner not interested in production boats, who wants to take part in designing their dream boat, it can be something of an ideal situation—provided they know what they’re in for.
Although it’s common for sailors to have a favorite build material, all options have their benefits and understanding their limitations helps to figure out which is best for each case. Perhaps the most important consideration when looking for a boat is the conditions it was designed for. Weekend race-boats are not designed for open-ocean conditions, nor are many cruising designs—even some of those that look the part. Unfortunately, finding reliable information for many designs can prove difficult, leaving owners to rely on their own judgement and on public opinion. To give a starting point for those interested in aluminum cruisers, the basics of some key design points are explored below. This content is simplified for clarity and isn’t intended for academic or professional reference—though I encourage the avid reader to use the citations provided to learn these points in proper detail.
BASICS OF ALUMINUM ALLOYS
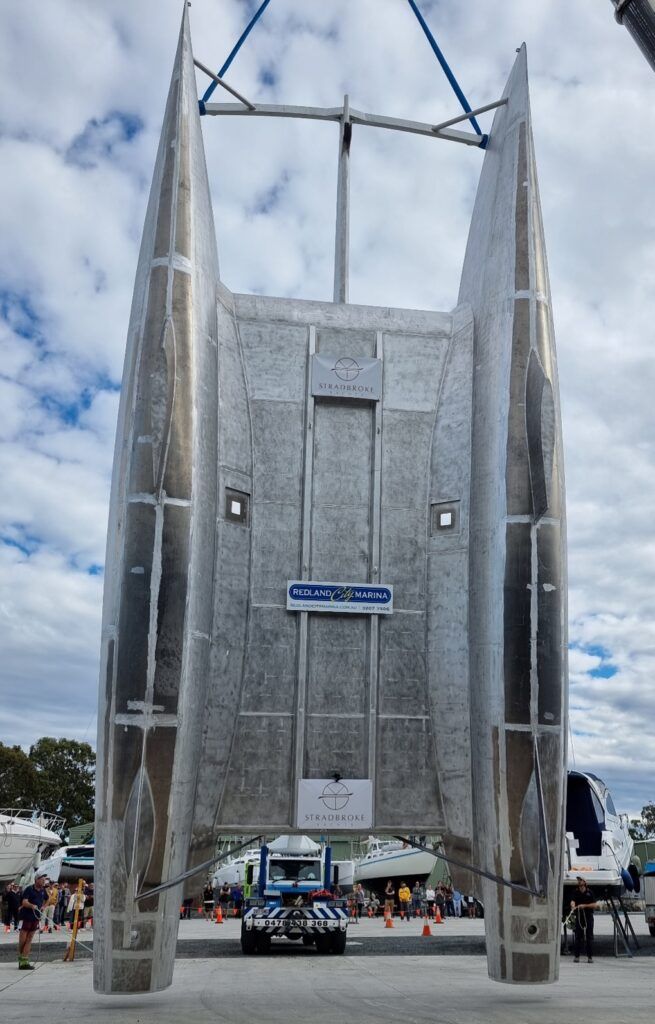
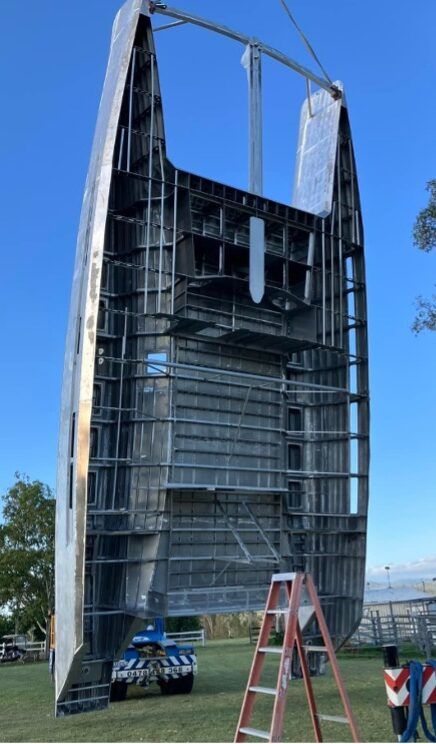
Despite using the generalized term of aluminum, the metals referred to here are not the one found on the periodic table. All are made with lesser amounts of various other elements to create an aluminum alloy, each of which are created and suited for different purposes.
The type of elements used defines the type of alloy, which are split into seven different series denoted 1XXX-7XXX. Each alloy type can then undergo further treatment, such as tempering or strain hardening, to improve its strength and other mechanical properties. Although this extra strength can be useful, the benefits are commonly lost once welded.
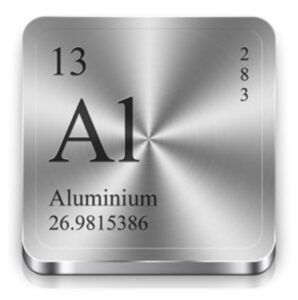
Modern vessels typically use 5XXX series and 6XXX series—each with desirable structural properties and corrosion resistance. Though better than many, the 6XXX is generally considered to have poorer corrosion resistance than the “marine grade” alloys in the 5XXX series. Because of this, boats built to a commercial standard have requirements for what grade is used in certain locations—a very good indication that cruising boats should follow suit.
Without a commercial survey or available build information, identifying the alloy proves a challenge. However, there can be some obvious clues. 5XXX is typically supplied in the form of sheets—the starting place of CNC cut structures. 6XXX alloys are a go-to for most extrusions; flat-bar, T’s, tubes and the like. When you are looking at an aluminum cruiser, identifying which parts of a boat are made from cut plate and which parts are extrusions will help estimate the alloy used, helping understand which areas are likely more prone to corrosion.
CORROSION: COMMON TYPES, IDENTIFICATION AND PROTECTION
Though renowned as a corrosion resistant material, in the marine environment this is still a significant concern, as if the conditions are right for it to start, it can progress at an alarming rate. Of the many types of corrosion that do occur in aluminum, three are particularly concerning: crevice, galvanic and electrolytic. All are related to electrical potential and are very similar in the way they work. In fact, the last two aren’t always considered separate “types” at all. A contributor to all three is an electrolytic fluid. Although this highlights the risk of damage around spilt Gatorade (or your other favorite sports drink), the more relevant fluid is salt water. Fresh water itself is an electrolyte, but a weak one—hence why this corrosion is a much larger issue on ocean-going craft than those on lakes.
Crevice corrosion. Crevice corrosion occurs where salt water is trapped and not refreshed in areas where it is let into the boat and left to sit. Two locations of particular concern are the rudder tube and stern tube (in which the bearings are housed), where stagnant seawater eats away at metallic rudder stocks and driveshafts on boats of all build materials. When the tubes themselves are aluminum these are even more prone to crevice corrosion, and particularly benefit from a corrosion-resistant alloy (5XXX series). Some commercial surveyors insist that a corrosion-resistant alloy be used in those areas.
As mentioned earlier, structure fabricated from plate is likely 5XXX series, so forming details provide a clue as to what grade has been used. A fabricated tube may be made from two rolled half-tubes, or from a solid piece of material with the hole bored. You may even see non-cylindrical tubes made from folded plate.
If extrusion is used, it’s nice to see increased thickness to allow for material loss. Even better to see is a detail that avoids contact between the aluminum and the internal seawater, such as the example in Figure 1.
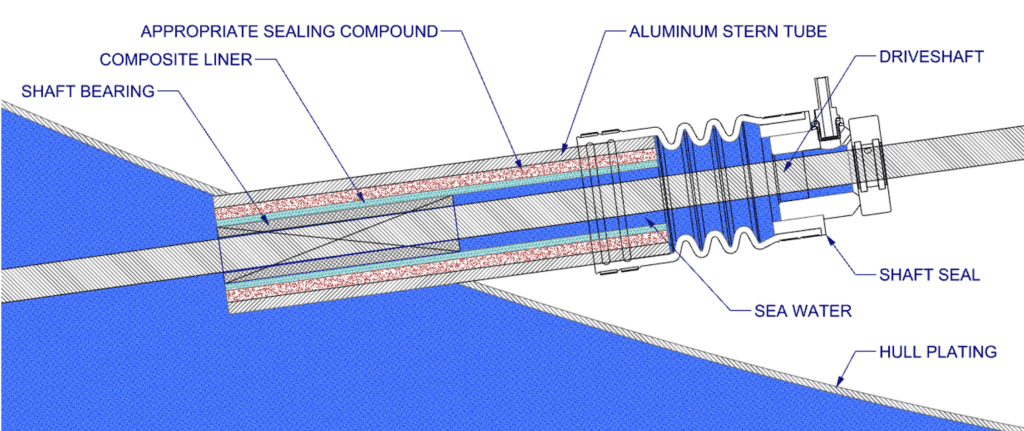
Galvanic corrosion. Galvanic corrosion happens where two dissimilar metals are in contact with an electrolyte—where two metals touch when submerged or in contact with salt water outside. Essentially this creates an electrical potential (a small voltage) driving a flow of electrons that break down the molecules of the metals, resulting in corrosion.
The key to understanding this is the galvanic scale, shown in Figure 2. Metals further to the left are more cathodic, while those to the right are more anodic. It’s the more anodic of the two touching metals that corrodes first. One of the furthest to the right is zinc, which is why it’s used to make the anodes found in marine supply shops. Aluminum alloys cover a relatively large range, which indicates the possible differences between the alloys. They are also relatively far to the right, showing their poor capabilities on this front and highlighting the importance of avoiding this situation.
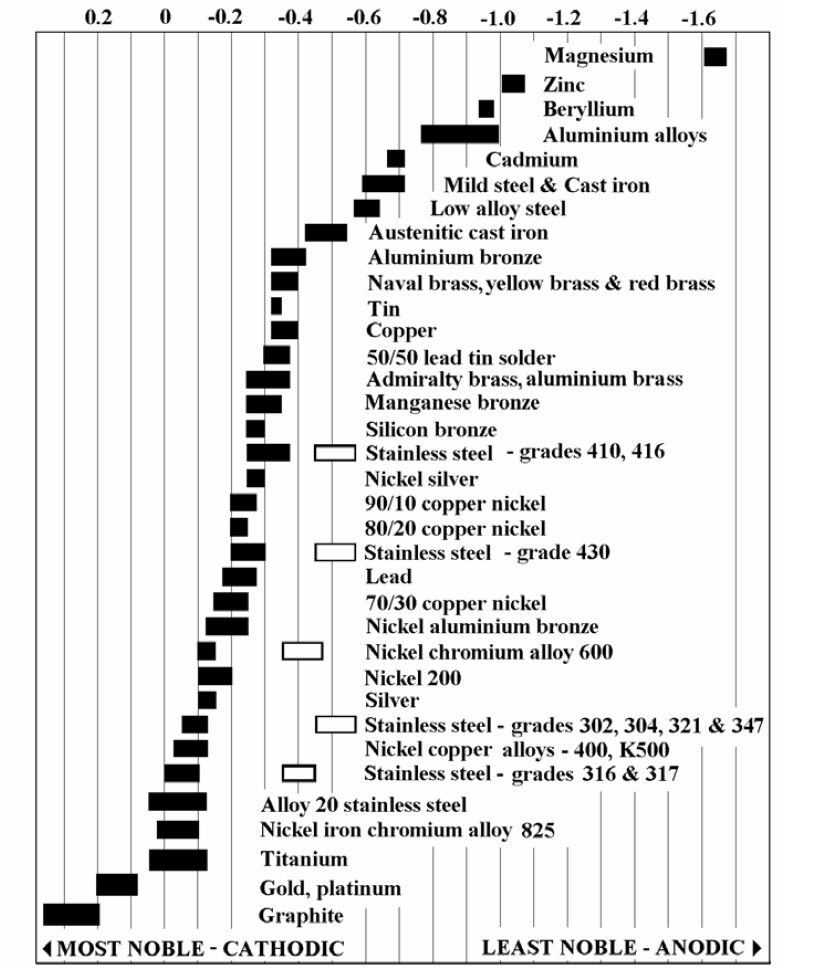
A common place for this to occur is at bolted connections, so isolating these as best as you can is key. Figure 3 shows an good strategy, with the bolt, aluminum structure and the item itself completely separated. If the item itself is aluminum, then the sealant between it and the deck is less important on the galvanic front, but it’s still important to prevent crevice corrosion. Anything non-conductive will act to separate the metals, and options such as sealants, gaskets and liners should consider the loadings expected. There are some specific anti-corrosive products on the market, such as Tefgel, that are worth looking into.
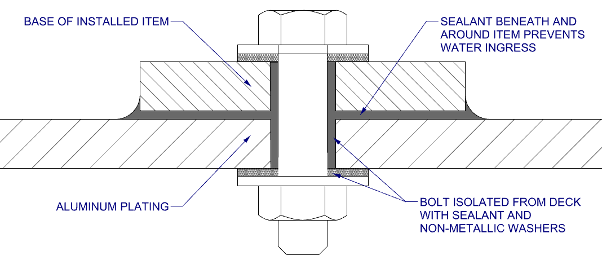
It’s also important to note the relationship between the two types of metal, as using a more corrosion-resistant material doesn’t necessarily mean galvanic corrosion will be reduced. The rate of galvanic corrosion depends on how far apart the two metals are on the galvanic scale, with a larger separation meaning a larger electrical potential and faster corrosion. Where two metals simply must be in contact with each other and with salt water—say for a bolted P-bracket—using aluminum-bronze fasteners should lead to slower corrosion than stainless steel fasteners.
Electrolytic corrosion. Electrolytic corrosion, also called electrolysis, is by far the most destructive of the three mentioned. Working on the same basis as galvanic corrosion, it is driven by an external voltage. Though usually from the boat’s electrical system, its surroundings are also a threat. High voltages, common from generators, inverters and shore power, allow the difference in electrical potential to pass through water and affect nearby boats—something to be especially aware of in marinas.
The fundamental protection method is through use of sacrificial anodes, which corrode before anything else, if installed correctly. There are debated approaches to what the correct installation method is, so I can only urge owners to go with a specialist opinion for their case—something that is generally provided with each new aluminum design. Noting their health is a great way to see if a vessel has an issue with electrolysis, and if so the first step would be to assess the onboard electrical systems.
Any point of electrical contact with an aluminum cruiser’s highly conductive structure can accelerate deterioration, so a healthy wiring system with well-covered and well-connected wires is a great deterrent. Non-conductive barriers between the hull and electrical equipment of significant voltage would presumably help also.
Provided these three types are prepared for, the corrosion issue is largely tackled. Fresh water makes it way inside through condensation and humidity, but it’s significantly less threatening than salt water—being a much weaker electrolyte. Nevertheless, it’s better to not let it accumulate, so drainage and ventilation are good practices. Longitudinal stiffeners are perfect for catching water, so having access to give them a clean up once a while is beneficial.
MATERIAL PROPERTIES: STRENGTH, STIFFNESS AND DUCTILITY
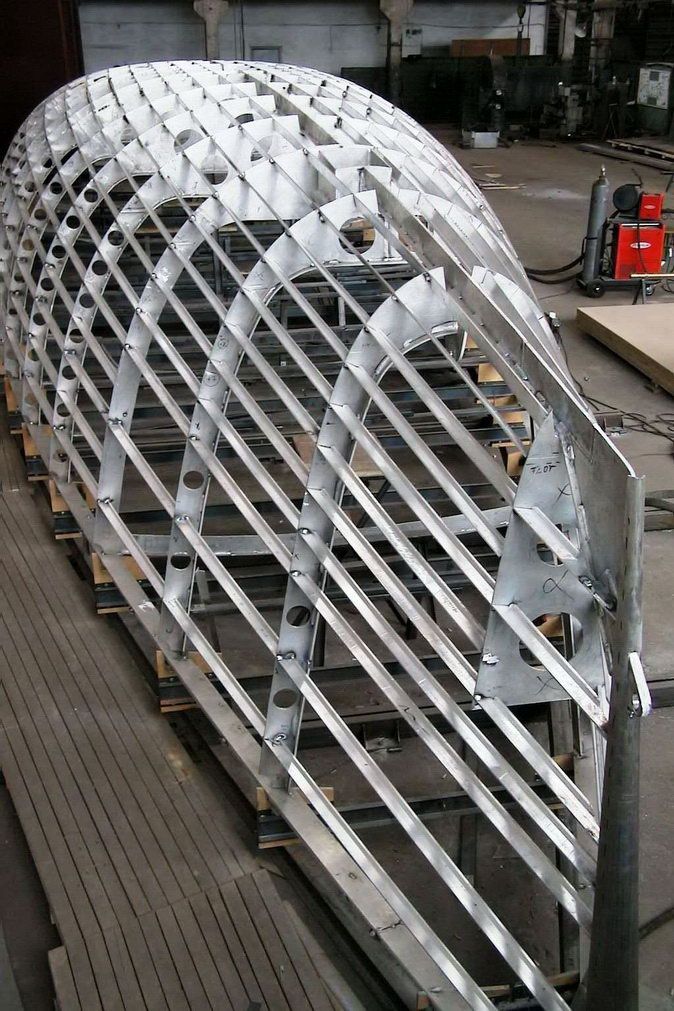
It’s easy to focus on strength when comparing build materials, but it’s important to realize the difference between a strong material and a strong boat. Each aspect of a boat should be designed to withstand the maximum loadings that it could see—regardless of the strength of the material itself. Steel is stronger than aluminum, but an aluminum cruiser can be built stronger than a steel one—it simply takes more material or a more effective structural approach. Similarly, a wooden boat can be built stronger than an aluminum one, despite being a significantly weaker material.
Considering two boats designed for the same purpose, we can assume each are comparably strong. However, when we don’t know these specifics (as is the usual case in the second-hand boat market especially), it can be handy to compare mechanical properties to relate back to a familiar material. This simplistic comparison can help explain the differences commonly seen in similar boats of different materials. It is by no means a thorough analysis—you should approach a suitably qualified engineer, naval architect or surveyor for that.
Table 1: Approximate Mechanical Properties of Boat-Building Materials
Material | Aluminum alloy 5083[a] | Corten Steel[a] | Plywood[b] | Fiberglass CSM[b] [N2] | Fiberglass Unidirectionals[b][N3] |
Design strength [MPa] [N1] | 125 | 310 | 21 | 85 | 1060 |
Stiffness (elastic modulus) [MPa] [N1] | 70000 | 200000 | 12400 | 6500 | 39000 |
Density [kg/m3] | 2700 | 7500 | 580 | 1500 | 1600 |
[N1]: to convert to imperial units, 1MPa = 145psi. [N2]: CSM = chopped strand mat. Laminated in polyester resin. [N3]: Properties are in the direction of the fibers, laminated in epoxy resin with high fiber volumes. |
A brief look at Table 1 shows that aluminum is not the strongest, stiffest or lightest option out of the compared boat-building materials, though it does provide a good middle-ground on these fronts. The first four options presented have been used for decades, by themselves, to build boat hulls, decks and cabins. Unidirectional fibers are a different story, generally used for reinforcement in specific areas. I’ve included these properties to highlight the large range in the material properties found in advanced composite designs, which make accurate generalizations about them near impossible.
Using the material properties of the first four examples, we can compare the required thicknesses of panels of similar same size to achieve the same strength. Figure 1 shows the material needed to match the strength of a 6mm aluminum panel to the nearest half-millimeter:

A key difference between these panels is their physical stiffness. Simply put, thicker objects are harder to bend, so the resulting stiffness of each panel changes despite the material’s “stiffness” (elastic modulus). Because of this, the plywood example is calculated to be over 2.5 times the stiffness of the aluminum, meaning it will deflect a lot less when a load is applied. Despite being a relatively stiff material, aluminum panels need a lot of supporting structure as their thickness is so low. Chopped-strand mat fiberglass isn’t a stiff material, but its thickness can easily be increased, leading to heavy hulls—hence why the majority of CSM boats are low-speed. For higher speed applications, fiberglass boats almost always use a sandwich laminate, increasing their stiffness and strength while maintaining a low weight through use of a light-weight core material as shown in Figure 5. This panel has about the same stiffness as 6mm aluminum but has over four times the bending strength.
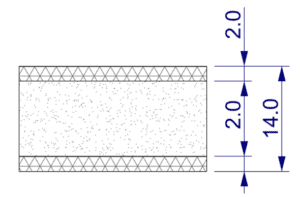
The point to note here is that simply stating that one build method is stiffer or stronger than another can be wildly inaccurate, especially where composites are concerned. I haven’t specified what type of fiberglass is used in this example laminate, as unless you have a boat’s build information, it can be hard to tell in a real world situation—leaving skin thickness as the main indicator of its properties.
A significant difference between materials is the way in which they fail. Fiberglass and wood are brittle materials; when they break, they break. Metals are ductile, bending—or yielding—before they break. As a permanently bent boat is not functional, designers typically base their calculations off the material’s yield strength rather than its ultimate strength, which for a go-to marine-grade aluminum alloy, 5083/H321, can be as much as 2.2 times stronger than the yield strength used for these comparisons.
Possibly the best feature of an aluminum cruiser is that if it hits a large, blunt obstacle (another boat for example), they very well may come out dented, but afloat. When vessels need repair in localized areas, the structure can often be cut out and new members welded back in. In the case of modern CNC cut vessels, the designer may be able to provide replacement parts to have on hand before cutting even begins.
STRESS CRACKING: CONCENTRATIONS AND FATIGUE
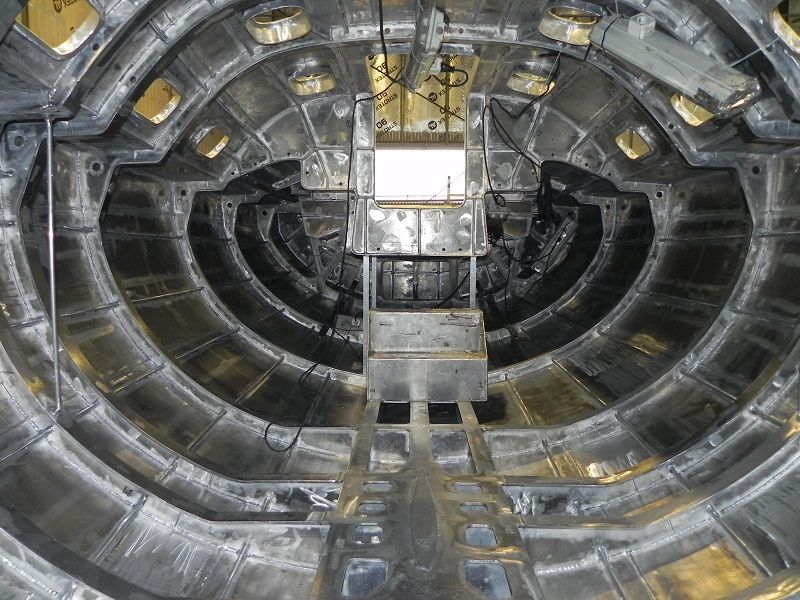
A challenge when designing aluminum structures is fatigue: the formation of cracks caused by cyclic loadings. Most loads applied to a boat are cyclic in nature; repeatedly applied as they move through waves and change speed and direction. Fatigue is a reality which can’t be avoided completely, but with diligent design it can be diminished to stretch the lifespan of a boat.
Cracking is mostly seen where the largest stresses are experienced and where strength is reduced. Abrupt changes in geometry, such as sharp corners or where two structural members meet, are known to have higher stress levels when under load. As mentioned prior, added strength from tempering or other processes is lost when welded—meaning the material’s strength is lowest at welded intersections. This combination means that the likelihood of fatigue cracking is highly dependent on the details used where frames and stiffeners (or other members) meet. To demonstrate these differences, the following images (created with Simscale’s FEA software) show how stress can be distributed across some common details.
Figure 6 shows two details where stiffeners pass through a frame. On the left, the stiffener is attached to the frame around the whole intersection, which results in higher stresses in both members—especially at the top. On the right, the time has been taken to include a stress-relief hole at the top of the stiffener, which is then attached only on its sides. As can the seen by the color differences, with this approach the stress is evenly distributed and is lesser in both members.
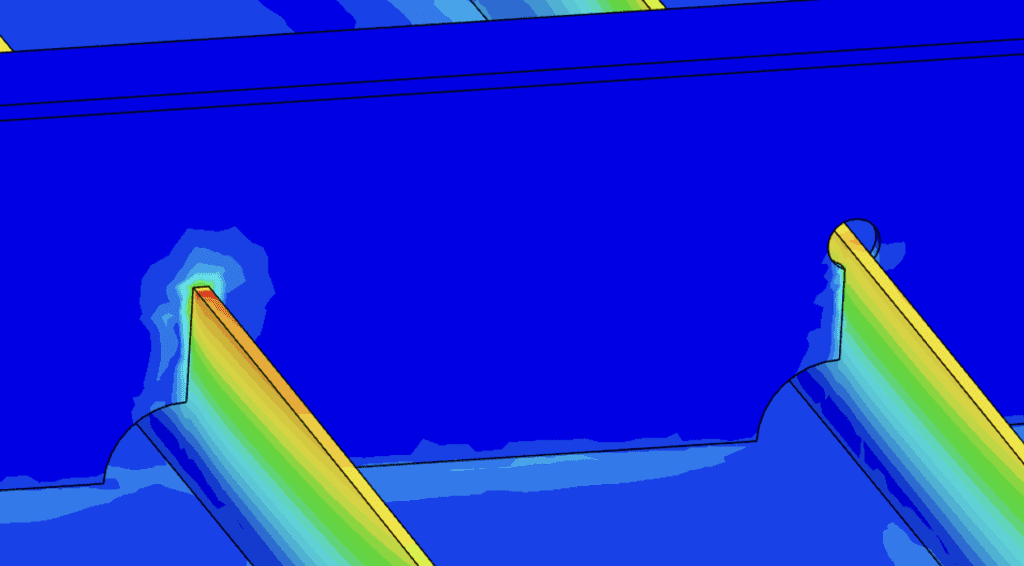
Figure 7 shows the stress distribution in a CNC cut stiffener with an integrated bracket (left) and one with a separate bracket welded on top of a flat bar stiffener (right). In both cases we can see the stress is highest at the base of each bracket, where there is the fastest change in geometry. Although similar levels of stress are experienced, note that in the right example this maximum stress happens at a weaker area (a weld), so there is an increased likelihood of cracking.
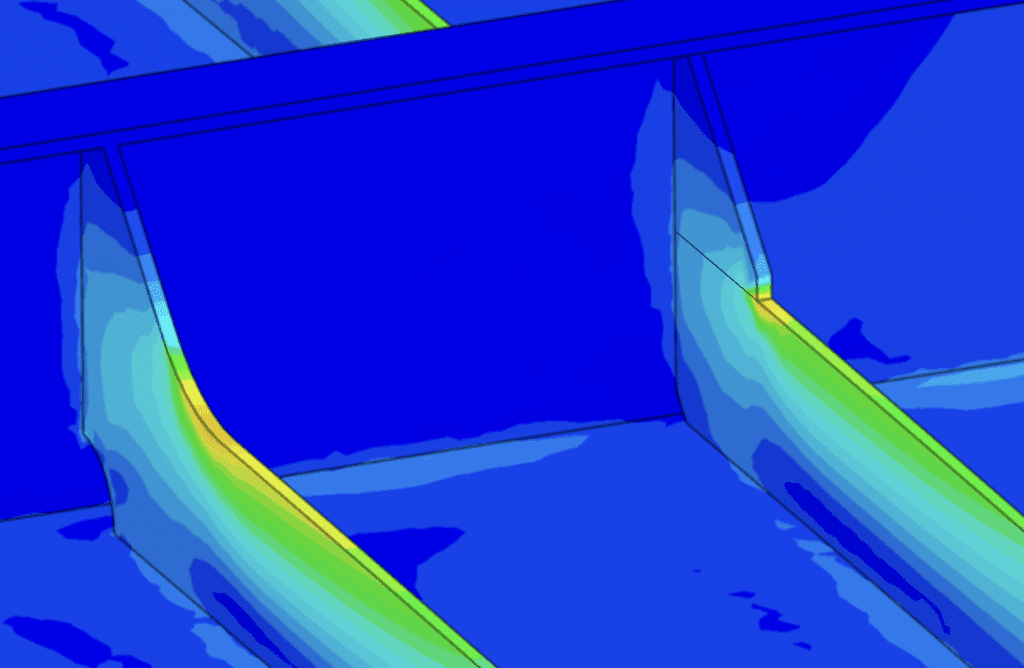
Given that this cracking occurs through use over time, it’s unlikely to be spotted on newer boats or less-used older ones. This doesn’t mean it’s not going to happen at some stage, so seeing stress-reducing details is a good indication of a boat with a long lifespan.
CONCLUSION
While this content should serve as a starting point of what to look out for, the best way to ensure all bases are covered is knowing the standard to which a boat was designed. If it has been assessed against a commercial standard, owners can rest easy knowing that a professional surveyor has approved the alloys and structural details used. Similarly, new builds with completely fresh electrical systems and professionally specified anodic protection plans significantly reduces the risk of corrosion issues. Although poorly maintained aluminum cruisers may steer buyers away, the market could soon be filled with a range of new custom and semi-custom vessels as more sailors explore the alternatives to composite production boats.
References
[a] Lloyd’s Register Rules for the Manufacture Testing and Certification of Materials 2020
[b] SSC-452 Aluminum Structure Design and Fabrication Guide