It’s been four and a half years since we last looked at carbon monoxide detectors (CO detectors). Since then much has changed with respect to the standards around which most detectors are developed. In the past there were two UL standards which governed the performance requirements of CO detectors. Marine/Recreational Vehicle and Residential were rated under separate UL standards. Today a revised standard exists which combines and expands requirements for all CO detectors. The structure of the new UL standard has had a positive influence on the sensitivity to and detection limits of CO. Today’s detectors are less likely to be overly sensitive in yielding nuisance alarms at low levels of CO.
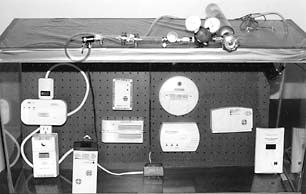
The new standard places a bottom detection limit where there was none before. When CO accumulation reaches into a range representing a true hazard, present-day CO detector alarms will sound, signaling a real hazard requiring a decisive response.
In this article, we’ll look at the hazards and dangers of CO as well as review and rate some of the detectors that are available today.
What is Carbon Monoxide?
Carbon monoxide was discovered in 1799 by English chemist Joseph Priestley. It’s a colorless gas or liquid, and is practically odorless. In concentrated form it is highly flammable. It is also highly toxic when inhaled, and has an affinity for blood hemoglobin that is over 200 times greater than that of oxygen. It’s also much less readily released from hemoglobin.
Although there are several ways to generate CO, it’s primarily yielded as a by-product of combustion. This is especially true of incomplete or partial (inefficient) combustion that takes place with a limited supply of oxygen (like an auto engine cylinder).
Just as a diver must be concerned with the buildup of nitrogen or oxygen in his blood from his air or nitrox gas supply, anyone breathing a mixture of air and carbon monoxide faces serious hazards.
Hemoglobin (Hb) is the respiratory protein of the red blood cell. It consists of 94% globin and 6% heme (molecular compound components). It transfers oxygen from the lungs to the tissues of our bodies while transporting carbon dioxide from our tissues to our lungs for expulsion. Each molecule of hemoglobin can combine with one molecule of oxygen to form oxyhemoglobin (HbO2). The heme portion of the molecule contains reduced (ferrous) iron, which enables the hemoglobin to combine with oxygen. This is one of the reasons why low blood/iron levels can produce symptoms of tiredness and fatigue as well as low stamina.
Because hemoglobin has such a high affinity for CO, any environment providing a CO supply will result in the greater generation of carboxyhemoglobin (HbCO) as opposed to oxyhemoglobin (HbO2). Although the carboxyhemoglobin is not toxic in itself, its formation is favored over oxyhemoglobin, resulting in the reduced transport of oxygen from the lungs to the tissues of the body. When high enough volumes of HbCO are formed, our bodies begin to suffer from oxygen deprivation in a process we know as asphyxiation. The greater the volume fraction of CO in the air supply, the more rapidly the body will form HbCO. At any concentration of CO, the percentage of HbCO in your body will continue to rise unless you are removed from the CO source. Once you’re removed, your body will take about five hours to cut its HbCO level in half. Because the body is so slow to rid itself of CO, the treatment of CO poisoning is much less effective than its prevention. This means that the CO detector plays an important role.
Symptoms
The symptoms of CO poisoning can be very subtle and progress slowly where low concentrations are concerned. With high concentrations, the onset of symptoms and death can result quickly. The progression of symptoms based on exposure level and time can be seen in the chart below. Other CO symptoms or signals may include tightness of the chest or hyperventilation, flushed face, fatigue or weakness, inattention or confusion, and lack of normal coordination. Drug or alcohol use may increase the effect of CO exposure and reduce the times shown below. People with either cardiac or respiratory conditions are very susceptible.
Even in a low-exposure environment, mistaking the symptoms for something else (such as the flu or motion sickness) and ignoring them can lead to unconsciousness and then death. Obviously, a CO condition generated at night when people are sleeping is extremely dangerous. The values of a reliable detection system are self-evident.
Boat Hazards
Burning fuels within or near enclosed or semi-enclosed spaces can result in CO generation and buildup. The fuels can include gasoline, oil, diesel, propane and other compressed gases, wood, coal, etc. Typically CO may exist wherever fuels are burned to generate power or heat. Burning compressed gases for cooking, heating (air or water), lighting, or cooling (refrigeration units) can also produce CO. Any situation where fumes may drift into the vessel also need to be considered, such as from auxiliary generators or exhaust backwash. Sources can even include CO from other vessels in your proximity.
A common situation exists where exhaust can be drawn into a cabin while underway. As air flows off the back end of any object past an abrupt transition, it will tend to vortex in the opposite direction. A stern or stern-quartering wind can make the situation even worse. Anyone who has motored for hours in a diesel-powered vessel in low wind conditions will remember the nagging odor of diesel fumes. If these conditions exist for a long enough time, they can cause a dangerous CO condition in a cabin or canvas enclosure.
During such conditions, close all aft portholes, hatches, etc., and open a forward hatch. No sleeping in aft cabins should be allowed while under power.
Windless nights can allow fumes to enter or congregate in cabin spaces. The use of exhaust fans can also draw in fumes. Caution should always be taken when at anchor or in a slip to safeguard against such conditions.
Detector Technology
No matter what sensor technology is used for the detection of CO, all detectors constructed for this purpose need to meet the UL spec. Basically there are two types of detection sensors used in these systems—tin oxide sensors and chemical sensors.
Tin oxide sensors are thick metal film semiconductors. They offer low cost, long life, and good sensitivity to target gases while using simple circuitry. The tin oxide sensor functions like a resistor, and in fact it’s the change in resistance that occurs from CO exposure that is detected.
In a typical environment with 21% oxygen, the semiconductor has a known resistance. When exposed to a deoxidizing gas, the surface density of negatively charged oxygen on the sensor surface decreases. This, in turn, reduces the resistance of the sensor. The change in resistance is compared to a known value stored in a microprocessor. The known value equates to a proportional CO level.
As changes in resistance and their corresponding CO levels are detected by the microprocessor, they are stored versus a time function. The exposure limits relative to time are known, and also stored in the processor. When the minimum acceptable levels are exceeded, the alarm will sound. The levels, as called out by the UL standard, are typically as follows:
70 (PPM) = 60-240 minutes (required alarm response time)
150 (PPM) = 10-50 minutes (required alarm response time)
400 (PPM) = 4-15 minutes (required alarm response time)
The response times specify a lower limit where there was none before. This has helped reduce the incidence of nuisance alarms.
Both the marine detector units we tested, the Xintex CMD-3M and the MTI Safe-T-Alert (Model 60-541) utilize this type of tin oxide detector sensor. Some of the RV systems that we did not test also use this sensor technology.
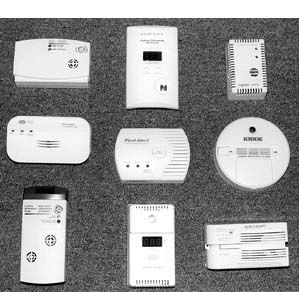
Some of the shortcomings of this technology relate to the sensitivity of the sensor to contamination sources. Exposure to silicone vapors like those used in silicone adhesives can damage the sensor. When these types of materials are used, the detector should be removed from the environment.
The sensor can tolerate large temperature swings if a thermistor is used to compensate for the output variation in the sensor. The thermistor yields a known resistance variation at a given temperature, which can be used by the microprocessor to compensate for the variation of the sensor. Most detectors using this sensor type use temperature compensation. Exposure to humidity swings will also have an effect on sensor sensitivity. Increased humidity yields increased sensitivity. Presently, no detector manufacturers compensate for humidity in their designs.
Direct contact of the sensor with salt water or condensation for a long period of time may result in a change or drift in the sensor characteristics. If the detector is to be stored, silica gel (as a drier) should not be used. It should be stored in a sealed contained filled with regular clean air. As the storage period becomes longer, a longer preheating period is required for the system to function properly.
Tin oxide sensor technology has been abandoned by some detector manufacturers, such as Kidde, in favor of the newer chemical sensor technology. The chemical sensor operates on a principle of a chemical reaction between a chemical medium and CO, generating a low-level voltage change due to a change in sensor conductivity.
This sensor technology is somewhat like a fuel cell. When CO is absorbed by the sensor, its conductivity increases. The amount of voltage on the sensor electrode increases in proportion to the amount of CO in the air. A microprocessor keeps track of the low-level voltage and its corresponding CO level in air to determine when the exposure limits are attained. At that point, the alarm sounds.
A relatively new variation on chemical sensor technology is to use a solid membrane as a sensor, instead of a gel or liquid system. Some of these types of sensors have a limited useful life, which can vary depending on the history of the exposure levels and times to which the sensor has been subjected. These sensors are also susceptible to contamination from various sources, including detergents, hair spray, paints or solvents near the detector, or the placement of the detector near any source of methane gas, such as directly above a diaper pail.
The Kidde detectors make use of a carbon filter bag located in the proximity of the detector air intake space. This acts to minimize the negative effects of small or subtle amounts of contaminants.
When Kidde first began using this type of sensor technology, the company had a recall on units that did not use the carbon filter bag system. It was found that outgassing from the polymer used in cover manufacture possessed enough residual contaminant (perhaps an organic solvent) that false alarms and readings resulted. Those problems have long since been corrected.
What We Tested, and How
A test chamber was made from a clean, 30-gallon glass fish tank. Highly pure carbon monoxide gas was precisely metered into the tank using a series of chambers to regulate and reduce the gas volumes to very low metered levels. The gas was introduced to the sealed tank through inert polymeric tubing connected to a football inflation needle valve. The tip of the valve was located immediately below a low-voltage computer chip cooling fan. The arrangement provided superb gas metering and mixing control within the tank.
We used a calibrated precision CO detector from the Fireboy/Xintex Company, which allowed us precise control of CO levels in the tank. Target CO levels could be achieved within 30 to 60 seconds from a zero reading and could be maintained indefinitely within approximately 2 parts per million (PPM).
We evaluated nine different detectors under the exposure and alarm guidelines called out in UL 2034. (Out of curiosity, we included an old 1997 plug-in Kidde Nighthawk.) We also ran a random ramp-up of CO and recorded the order in which each detector alarmed.
The detectors were placed in the test tank filled with regular atmospheric air. After prescribed warm-up periods, CO was metered into the sealed tank until required levels were reported on the calibrated precision detector. A timer was used to determine the response time of the detectors that were tested. Upon alarm of the last detector, the test was stopped. The volume of the tank was replaced with clean air and the time-to-silence was checked. All systems were depowered and allowed to sit in fresh air for 24 hours prior to testing at the next level.
During the test runs we evaluated the effectiveness of the reset and silence features where provided. The results of our tests can be seen in the chart on page seven.
Performance Discussion
All detectors performed within the UL-specified response windows (with the exception of the old Nighthawk—see chart). We want to state clearly that only the Fireboy/Xintex CMD-3M and the MTI Safe-T-Alert 60-541 are approved under the new standard for marine use. Also note that they’re the only 12 VDC models. Their manufacturers recommend that they be hard-wired and permanently powered.
It should also be noted that under UL 2034, marine-approved detectors need to undergo several special tests that other household type units do not. They include shock testing (5,000 cycles @ 10 Gs duration of 20-25 milliseconds/event), large humidity swings, vibration, drip test, cold temperature exposure, and a host of other requirements. These tests are designed to replicate some of the worst conditions a marine detector might be subjected to while a vessel is in operation. Home detector units do not have to meet the same performance tests as either marine or RV units, although many times home units are used in both. We were interested to see how the home units stacked up against the marine units.
Three of the detectors we tested were battery units, four were plug-ins and two were 12-volt DC hard-wired. Three of the four plug-in units we tested had LED readouts of CO PPMs.
Our old Nighthawk was reading low at 70 PPM with values of 12 within one minute. The American Sensors unit read consistently high at 105 PPM after one minute. The new Nighthawk model read superbly over all ranges of PPM. It generally read slightly higher than actual values but responded quickly to increases and decreases in CO level with accurate readings. At 400 PPM exposure levels, the new Nighthawk read values of 469 after two minutes with the American Sensors unit signaling 999 PPM. The Kidde Nighthawk unit was the most accurate and responsive LED detector we looked at.
The Nighthawk had other nice features, like a peak level button that displays the peak CO level detected since the last reset. Both the new Nighthawk plug-in and its battery-operated cousin yielded some of the longest response times to alarm condition. At 70 PPM they were second only to the Xintex marine unit in waiting to alarm.
We assume here that it is more desirable for the detector to alarm towards the long end of the window allowed by UL. This translates to slightly less sensitive detector/alarm thresholds used by both Kidde and Xintex. Performance like this would be less likely to sound nuisance conditions that may take care of themselves in an acceptable period of time. This may be less desirable to some people, who would instead favor the Canadian-built American Sensors model CO1100G, battery-powered. At all levels of exposure we tested, this unit responded to CO the quickest. The First Alert battery powered unit (FCD3N) was also fast to respond at all levels of exposure.
Conclusions
Of the two marine-approved units, the Fireboy/Xintex (CMD-3M) and the MTI Safe-T-Alert (60-541) the Fireboy model was our tester’s choice. This unit is simple, rugged, and cost-competitive with the MTI unit.
Our decision was influenced by the fact that the MTI unit’s low-level alarm would ring considerably earlier than the Xintex unit. Resetting would silence the unit for a few minutes, but another alarm would follow. At a later point, the LED that lights up in the alarm mode simply becomes solid red, as opposed to flashing to signal a higher CO level alarm.
The MTI has other positive features, like the ability to operate without a problem over a wider voltage range. This means that as batteries become weak, the MTI unit can continue to operate longer than the Xintex. However, the Xintex unit does have a unique low-voltage alarm mode to signal the condition. The MTI unit will eventually signal a malfunction, with a suggestion to replace the unit. The MTI also offers a memory feature that the Xintex does not: After an alarm, the MTI can “play back” the exposure level causing the alarm by using a series of beeps and red LED flashes. Remembering what each means can be difficult, but a handy sticker on the top of the case is there to remember for you.
We like the additional features of the MTI Safe-T-Alert, but feel that the low-level alarm is not necessary. An alarm in the middle of the night is still an alarm, whether low- or high-level. The Fireboy/Xintex has fewer features, but will get you up when it really counts. When it alarms, there’s no question about the exposure situation. Again, some would appreciate the earlier warning of the MTI. They are both excellent units.
We also really liked the Kidde Nighthawk units, especially the LED. Perhaps the LED display is not shock-tolerant enough to apply to marine units, and perhaps the marine/RV market is not considered large enough for Kidde to play in; but their units would be welcomed if they were designed to meet the Marine/RV specs.
With respect to cost, numbers have come down some since our last report. The battery-operated units fall in the $20 range, the plug-in models in the $34 range, and our two marine units in the mid to upper $50 range. Four and a half years ago, they were in the $70 to $80 range, which was a third less than five years previous.
A final reminder: Some new boats have a significant enough level of monomer and solvent outgassing upon delivery to cause false alarms in most of these units. It may take a couple of months before this problem abates.
Also With This Article
Click here to view “CO Detector Performance.”
Click here to view “CO Poisoning Symptoms.”
Contact – American Sensors, North American Detectors, Inc., 2600 John Street, Unit 15, Markham, Ontario, Canada, L3R 3W3 416/493-9169, www.nadi.com. Fireboy-Xintex, P.O. Box 152, Grand Rapids, MI 49501, 866/350-9500, www.fireboy-xintex.com. First Alert Inc., 3901 Liberty Street Rd., Aurora, IL 60504, 630/851-7330, www.firstalert.com. Kidde Safety, 1394 S. Third Street, Mebane, NC 27302, 800/880-6788, www.kiddesafety.com. MTI, 31632 N. Ellis Dr. #301 Volo, IL 60073, 800/383-0269, www.mtiindustries.com.