Photos by Ralph Naranjo
When a keel tears away from a sailboats hull, it makes the loss of a rig or rudder seem like a minor inconvenience. History shows that its an uncommon occurrence, but because we now annually hear of such incidents, weve decided to take a closer look at keels and see what keeps the ballast where it belongs.
The International Sailing Federation (ISAF) Offshore Special Regulations devotes pages to helping sailors prevent and respond to a crew overboard incident. There is nothing about how to handle the loss of a keel or ballast bulb. Some might say this is because such occurrences are so infrequent, while others note that, if youre still upright once the ballast breaks off theres not much you can do other than blow the sheets, douse the sails as quickly as possible and attempt to stop any leaks.
When solo sailor Mike Plants Open 60 Coyote lost her lead bulb in 1992, Mike was lost at sea. Other adventure-sailors have survived near instantaneous capsize precipitated by keel loss. In 2003, round-the-world racer Tim Kent and his crew capsized when Everest Horizontal lost its ballast on the way back from Bermuda. US Sailing Safety at Sea Committee Chairman Chuck Hawley was aboard the racing sloop Charlie, on the way back from Hawaii, when a loud groaning sound led to a deep heel as the lead peeled away from the keel bolts and ballast headed straight to the bottom. This encounter at least had a happy ending thanks to the crews quick actions to douse sail. Apparently the keel had been cast with too little antimony (an additive that causes lead to become a harder alloy). The point here is that keeping the keel attached is as important as keeping the crew safely on board. And for the offshore monohull sailor, preventing a keel loss, like preventing crew overboard, requires some informed forethought.
A ballast keel on a sailboat is a classic example of potential energy poised in a balancing act. The buoyancy of the hull itself offsets the effect of thousands of pounds of lead or iron. At rest, gravitys attraction for the dense material strains against the buoyancy of the hull, and the adjacent garboard region is continuously in tension. Few sailors spend much time contemplating how keel bolts corrode and what cycle-loading does to the resin matrix comprising the garboard region just above the ballast. What is apparent, is that the attachment material, whether it be wood, metal or fiber reinforced plastic (FRP), must be able to support a mass of metal weighing as much as a small truck-and do so day in and day out for decades.
Underway, every tack causes the rig and sailplan to try to lever this ballast package free from the hull. And when the helmsman starts daydreaming about lobster for dinner and wanders off course onto a granite ledge Down East, the keel designed to handle sailing loads takes it on the chin. Its easy to see why experienced designers and builders lose sleep over their decisions about keel shape, structure, and what kind of safety factor should be built into the structure.
Its surprising to discover that with better materials and computer-aided design, we still hear about incidents such as the Rambler capsize in the 2011 Fastnet Race (PS, May 2012). Just as significant is a spate of smaller race boat keel-ectomies that have caused ISAF to send out a cautionary note to sailors around the world, and introduce new structural standards for race boats. Keeping the ballast attached to the boat involves an awareness of a chain-like set of failure points. And one of the most difficult decisions each designer must make is how to marry foil efficiency with a structural safety margin that covers the boats intended usage and the unintended use of the keel as a depth sounding device.
For decades, engineers and naval architects have had to contend with some racing sailors Icarus-like quest-a trend that prioritizes shedding weight and making the keel foil a long, thin appendage with a high-aspect ratio. Though not quite a flight toward the sun with wings made of wax and feathers, some race-boat scan’tlings walk a fine line between lightweight and structural failure. The challenge lies in attaching a lead bulb on a high-tensile steel foil to a lightweight, high-modulus, FRP hull. Interconnecting the dense metallic ballast to the lower-density foam/fiberglass hull structure is a true engineering puzzle. Part of the challenge lies in the dissipation of point loads (confined to a relatively small area) and how to handle the resulting stress risers. A stress riser is the point at which theres an abrupt change in a materials flexibility, such as where a stiff, fin keel meets the more elastic hull bottom. In FRP composites like those found in a balsa-cored hull, stress risers are a likely place for delamination to occur. Over time, these can result in the failure of the FRP composite.
The see-saw effect of the keel counteracting a vessels righting moment is a mathematically predictable energy transfer. Even the effect of groundings such as those that turn hull speed into a dead stop can be quantified. But its the cumulative effect of fatigue (localized structural damage caused by cyclical loading) and corrosion that are harder to pin down.
The term allision refers to hitting a fixed object such as a granite ledge or coral reef. Naval architects analyze the energy transfer and evaluate the stress and strain characteristics that occur. The recognition that the keel-to-hull connection must endure even more punishment than is doled out in heavy-weather sailing episodes is at the heart of how structural specs are devised.
Designers also must consider the jack-hammer-like pounding of a keel on a reef in surf, and realize that there are limits to the abuse a keel and hull can endure. With this in mind, its reasonable to assume that sailboat keels should be built to handle sailing induced loads for decades. It is the extra safety factor built into the boat that defines what happens when the sandbar is a rock pile.
What is harder to anticipate are the unusual encounters that can inflict serious damage to the keel connection. Take, for example, what happens when a sailboats deep fin keel is wedged in a rocky cleft and a good Samaritan with a big powerboat attempts to pivot the sailboat using a line attached to the bow. The distance from the keels vertical centerline to the stem may be 20 feet or more, and with a couple of thousand pounds of bollard pull, the 20-foot lever arm creates a rotary force that can spike to 40,000 foot-pounds or more. This level of torque goes well beyond what most designers and builders model as sailing loads, and its likely to seriously damage the boat.
In plain low-tech talk, extreme fin keels provide a valuable performance edge, but they come with their own set of downsides that every owner needs to be aware of. In essence, the more radical the keel shape, the better the crew must navigate.
A couple of decades ago, PS Technical Editor Ralph Naranjo ran a boatyard and had a client who liked to cut the corners during Block Island Race Week. His first spinnaker reach into a granite boulder stopped the boat and shoved the companionway ladder upward six inches. This underscored how an allision that causes the keel to stop abruptly transfers a shock wave through the entire hull. The resulting compression cracked several transverse members in the New York 40 and damaged the core in the canoe body near the garboard.
The FRP repairs had to be tapered and all delamination problems resolved. The moderate-aspect-ratio lead fin keel absorbed a good deal of the blunt trauma. Judging from the cannonball-size dent on the leading edge of the lead keel, it was clear that the impact was significant. The dent offered grim proof of the advantage of having soft lead instead of steel as keel ballast. New floor frames were added, the broken transverse members were replaced, and the boat was off and sailing.
The next season, the boat had another Block Island encounter, and only because the Petersen-designed New York 40 was a pretty ruggedly built boat was a second repair even considered. This time, an equally violent keel-to-hull trauma came from an on-the-wind encounter with a different rock. The extent of the delamination was greater than it had been in the first go round, and more extensive core removal and repair was required. The keel was dropped in order to check the bolts and the garboard. With the bilge fully opened for the FRP repair work, the repair crew made a pattern of the canoe body dead rise and fore and aft contour. As the glass work was being completed, they fabricated a stainless-steel grid that would spread keel loads fore and aft as well as athwartship. The new grid reinforced the keel attachment and returned the sloop to the race course.
Afterward, Naranjo and the owner discussed the details of the repair, including the possibility of hidden, widespread damage from the two groundings. These included the dynamic loads imposed upon the chainplates and rigging, the likelihood of hidden resin-cracking, and potential for more delamination and core shear linked to the torque induced by the accident. In short, any serious allision causes overt and hard-to-detect damage far from the actual impact zone, and these can lead to more problems down the road. When buying a used boat, look for a good pedigree, but also look for signs of previous blunt-force trauma. A good surveyor will be skilled in such structural forensics, and he or she will do more than comment on the gelcoat shine.
Keel 101
In the early days of wooden ships and iron men, a lack of dense metal ballast put less point-loading in the garboard region of the hull. Bilges free of cargo were filled with rocks or tighter-fitting granite blocks cut for more compact stacking. The principal of ballasting a vessel was to lower her center of gravity (CG) and create both an increase in the righting arm and a greater righting moment to offset the heeling moment created by the rig and sail plan. The keel also helped lessen leeway and would evolve into an appendage that added lift.
Movable ballast had a few downsides, not the least of which was its propensity to move in the wrong direction at the very worst moment. Even small boat sailors have found out what can happen to unsecured pigs of lead ballast when the boat heels far enough over for gravity to overcome friction. Whether stones, lead, movable water ballast, or a can’ting keel are used to augment the boats righting moment, a sailor must anticipate the worst-case scenario. This is when the weight ends up on the leeward side of the boat and a bad situation can turn into a real catastrophe. Fixing or locking ballast in place, controlling the volume of water put in ballast tanks, and limiting the can’ting keels range are sensible compromises.
Internal ballast, the ballast inside a keel envelope thats contiguous with the hull, is still seen in many new boats. Island Packet is an example of a builder has stuck with this traditional approach of securing ballast without using keel bolts. Its a sensible design for shoal-draft cruisers, and the upsides are numerous. These high-volume, long-range cruisers arent encumbered by the demands prioritized by light displacement, performance-oriented sailors. Instead, Island Packets combine a rugged laminate and a long-footed, shallow-draft keel. This may not place the lead or iron ballast as deep as the tip of a fin keel, but it does keep the all-important CG low enough to deliver a powerful righting moment along with shoal draft.
In order to deliver the high angle of vanishing stability (AVS) also known as limit of positive stability (LPS), designer Bob Johnson puts what amounts to an internal bulb in the very lowest point in the boat. This long slug of iron or lead (depending on the model) is then covered by Portland cement, locking it in the Island Packets monocoque structure. The result is a contiguous FRP structure spreading keel loads efficiently over a considerable amount of hull skin. Keel bolts and the infamous garboard seam are completely eliminated. This approach to sailboat keel design dates back to the Rhodes Bounty II and other prototypes in the production world of sailboats. Now over 50 years old, many of these boats continue to have a tenacious grasp on the lead or iron that they hold.
Encapsulated iron ballast is much less desirable than encapsulated lead, and its sad to see builders skimp on this. Iron, or even worse steel, has been used in many Far Eastern encapsulated keels. It works as long as water and the resulting oxidation havent caused expansion and cracking of the seal. Lead is also denser than ferrous metal, and therefore, the same amount of ballast will have a smaller volume and create less drag.
Encapsulated ballast starts to be less appealing as keels become more fin-like and high-aspect ratio. The reason for this is that the geometry of the support changes, focusing more load on less area of the hull. As hull shapes evolved into canoe underbodies with hard turns in the bilge, and fin-like keels became thinner, deeper, and with shorter chord measurements (thickness), the concept of encapsulated keel became impractical. The Cal 40, Ericson 39, Pearson 365, and a long list of similar genre boats signified the end of an era when performance racer/cruisers would be built with encapsulated ballast.
External Ballast
Performance-oriented sailors and race-boat designers quickly latched on to hull shapes marked by deep-draft, foil-shaped, high-aspect ratio fin keels. From the late 60s to whats currently glowing on CAD screens in designer offices around the world, keels have grown deeper and shorter in chord length, and bulb or anvil-like tips have grown more and more common.
The design development was sound, lift was enhanced, and deeper-not longer-became the answer to getting to windward faster. The challenge was not only in designing an efficient shape, it lay in creating an attachment means that minimized foil flex and twist, retained the low drag coefficient, and still had the ability to withstand an occasional, albeit modest, grounding.
During this same period, marine surveyors and boatyard techs began to see moderate groundings result in major structural problems. The classic example was the allision that produced a moderate dent in the lead at the leading edge of the keel tip. In many cases, further inspection revealed cracks radiating outward from a knot meter or depth sounder mistakenly placed just ahead of the keel. An even closer look often revealed grid damage or a cracked bulkhead just aft of the last keel bolt. Like the New York 40 mentioned earlier, this was a result of a shock wave radiating through the hull structure. As we learned in Mrs. McCrearys science class, Bodies in motion tend to stay in motion, unless acted on by an equal and opposite force. Fin keel sailboats encountering abrupt energy transfers,tend to endure more damage than their long-keel counterparts.
A forensic look at the Achilles heel of external ballast highlights a few pitfalls. First the good news: Lead absorbs impact well, consuming much of the imparted energy through deformation. However, the translation of the remaining energy from the metal keel foil and keelbolts into an FRP hull is where we often find stress risers, and point loading linked to material and hull shape changes. The near right-angle interface between a modern sailboats canoe body and its deep fin keel is a classic load-path hotspot. In the old days, fiberglass techs spoke of oil-canning or the dimpling of a large section of the garboard as tacks were swapped.
Today Naval Architects use Finite Element Analysis (FEA) to better engineer hull structure. Colorized graphics pinpoint load concentration, glowing bright red in the region where the keel joins the hull, the epicenter of the oil-canning. A common solution to coping with this high-load focal point, is to eliminate core in the region and to gradually increase the unit schedule (layers of FRP), or to add an internal FRP grid. Maximum thickness of a keel stub is located where the keelbolts penetrate the stub. In this region, the solid glass thickness is often equal to the dimension of the keel bolt diameter or even greater.
Laminate thickness at the keel bolts is only part of the equation. Just as important is how the transition to the general hull laminate transpires. A bullet-proof keel stub that immediately transitions into a core hull comprising two units of laminate on each side of the panel creates whats equivalent to a tear-on-the-dotted-line weakness. Transitions that involve sharp angles and marked differences in panel strength require a well-reinforced taper that spreads loads gradually rather than abruptly.
Occasionally, we see massive metal frameworks used in the bilge as support for keel bolts; these structures need to be carefully engineered to not create the same hard spot fracture points. When carefully tapered in order to gradually introduce more flex, the problem is abated, as it was in the repair of the New York 40 mentioned earlier. The stainless-steel grid built to support the keel loads incorporated a gradual decrease in stiffness to the framework. The keel was carefully mated to the underside of this grid to ensure full contact (See Keel Bolt Repair Options, online). As a result, the crew relieved the hard spots at the end points and made the transition to the more flexible FRP hull less dramatic.
Conclusion
For cruisers, the take-away lesson is that extra reinforcement, a long garboard keel-to-hull interface, and internal transverse and longitudinal reinforcement really do pay off. Keep in mind that the extra weight this entails is all below the center of gravity and contributes to the secondary righting moment as well as keeping the water out.
This is a big departure from the way many modern production boats are built. They carry a skimpy ballast ratio of 30 percent or less, have less structure to support the keel and are not designed to handle unintended cruising consequences. There are exceptions, and its worth looking at the keel design and structure of the Navy 44 Mark II and the USCG Leadership 44 (see PS, August 2012). These boats utilize external ballast and are examples of rugged keel attachment. They have a relatively long keel-to-stub garboard junction, the laminate scan’tling meets American Bureau of Shipping recommendations, and both utilize an overabundance of 316 stainless-steel keel bolts and an FRP grid to keep the keel where it belongs.
There are many reasons why were seeing more keel problems today. On one hand, light, fast, race-boat design pushes the envelope, and thats probably OK. But when mainstream racer/cruisers start to suffer from lead loss, too much of one good thing (high-aspect ratio) and too little of another good thing (reinforcement) can begin creeping into design and construction.
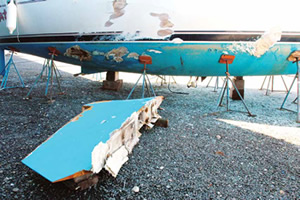