by Nick Nicholson
Most of us have boats built of fiberglass, laid up in female molds as part of a production boatbuilding process. We have a vague idea that the hard outer surface is made of gelcoat, that the reinforcing material is probably glass fiber, and the resin used is most likely polyester. Beyond that, our knowledge of the boatbuilding process approaches zero.
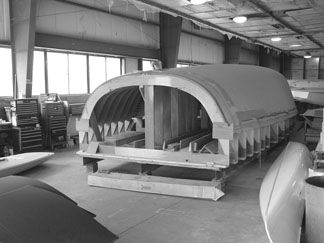
In small shops around the world—sometimes converted warehouses, sometimes surgically-clean purpose-built facilities—custom boatbuilders test the boundaries of technology in a way that no production builder can. They may speak English, Italian, Spanish, or Swedish but they share certain common characteristics: a lack of fear of the unknown, complete inability to say “it can’t be done,” and an insatiable desire to build boats better than anyone else in the world.
And, yes, a lot of them feel that nobody—but nobody—can build boats as well as they can.
In boatbuilding, as in most endeavors, the state of the art is a moving target. Today’s one-off innovation is incorporated into tomorrow’s mass-produced product. This month, we look at some of the characteristics of boatbuilding on the edge—the methods and materials used by the best builders and designers working today.
Lofting and Tooling
While female tooling—a concave mold whose inner surface replicates the outer surface of the component molded in it—has been the norm for series-produced boats since the late 1950s, it is, ironically, relatively uncommon at the cutting edge of boatbuilding technology.
The reason is cost. Boatbuilding is a multi-stage process. Whether the goal is to produce a single hull or 500, the first step is to construct a male mold, or “plug,” which represents the finished shape of the hull. If a female mold is to be made from the plug, the plug is identical in shape to the finished hull. If a hull is to be molded directly on the plug without creating female tooling, the plug is the shape of the boat less the thickness of the finished hull itself.
Think about it. The plug is created by using the designer’s sets of two-dimensional hull lines to create a three-dimensional matrix that defines the shape of the boat. In the good old days of composite boatbuilding—say 25 years ago—the hull lines were presented to the builder in the form of a table of offsets at defined distances from an XY datum. The builder carefully plotted these offsets full-size on the lofting floor, fairing between points with a flexible batten. Patterns of the hull sections were then taken from the full-size drawing to serve as forms for the construction of the plug. Clearly, this is a labor-intensive process requiring skilled labor.
Lofting changed little from the establishment of scientific naval architecture several hundred years ago to the development in the last two decades of computer programs that freed the designer and builder from the tedium of hand-drawing of hull lines. Now, the hull lines are created in a computer program, and converted directly into numeric data files that can be used by a computer-driven machine to cut the hull sections used to create the mold.
The hull shape is still the product of the designer, but he uses computer tools rather than pencils, paper, and a set of drawing curves. There is still art in the process, and the best computer program in the world will not turn Joe Blow into Nathanael Herreshoff.
In production boatbuilding, the male plug created from the hull lines serves as the form for creating a female mold which will ultimately be used to lay up the hull. For one-off boats or the production of a small number of hulls, the hull of the boat is molded directly on the male plug. The outer surface of a hull created on a male plug must be faired and painted, as it will not be as smooth as the outer surface of a hull laid up in a polished female mold.
At the cutting edge of technology, the male plug is machined from a rough form covered with high-density foam. In many shops, a huge five-axis milling machine, driven by a computer program containing the hull lines, carves the finished outside hull shape from a rough block with remarkable precision. This is actually a lot more complex than it sounds because the foam surface must be reinforced to make it hard and smooth enough to be used as a plug for making the female mold. Whether the male plug is built by hand or carved by a milling machine, the female mold for a production fiberglass sailboat is made directly on the male plug using conventional gelcoat, fiberglass fabric, and polyester resins. At the cutting edge, however, state-of-the-art boatbuilding takes an expensive turn.
America’s Cup boats are built to a remarkable level of accuracy, where a hull that diverges an eighth of an inch from the designed shape can result in heated discussions between designer and builder. Traditionally—if such a term can be applied to a class that is little more than a decade old—the hulls were laid up on a male plug, then filled and faired to the final hull shape using templates. Even the most carefully-built boats might have several hundred pounds of filler, primer, and finish paint on the surface in order to achieve the shape created by the designer.
In order to reduce the weight of the hulls, syndicates with the deepest pockets now go the extra step, creating female tooling for one-off boats. All the fairing effort goes into building the plug used to create the female mold—unless the plug is computer cut, in which case no fairing is required. But this isn’t the end of spending. It is critical that the designer allow for the changes in shape that the hull and tooling will go through during the heating process that cures the hull. The only way to make sure that the hull and mold will contract and expand together without distorting the hull shape is to make the female tooling of carbon fiber, just like the finished hull itself.
Effectively, two carbon-fiber hulls must be built—the mold and the hull—to end up with one finished female-molded carbon-fiber hull. When the carbon-fiber hull is removed from the female mold, the hull surface is perfectly smooth—no filling and fairing required. A light coat of sprayed finish—note that many of these boats are black—protects the epoxy/carbon hull from ultraviolet light, which would degrade the epoxy.
Reinforcement Fabric
The standard reinforcement material for state-of-the-art sailboat construction is carbon fiber. As anyone who owns a carbon fiber spinnaker pole can tell you, carbon is light, stiff, and strong. Like glass fiber, it can be woven into cloth, held together in parallel strands, or be generally assembled into materials with any number of combinations of fiber orientation and weight. Just as in fiberglass construction, these layers of carbon “cloth” are layered in the female mold or on the male plug to create the hull skin. The position of each piece of reinforcing fabric, its weight, and its fiber orientation are carefully engineered to optimize physical properties and minimize weight.
Resins
Polyester and vinylester resins are widely used in production boatbuilding. Polyester resins have been the staple of composite boatbuilding since day one, thanks to their relatively low cost and ease of catalyzing. Vinylester resins are often used in the outer layers of production composite boats due to their greater resistance to osmotic blistering when compared to most polyester resins.
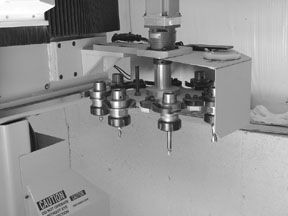
In state-of-the-art building, however, epoxy resins dominate. Their greater mechanical properties blend well with the high-tensile carbon fibers normally used in this type of construction, more than offsetting the higher cost of the epoxies. One of the great leaps forward in construction has been the use of fabrics pre-impregnated with resin. In conventional construction, the reinforcing fabric is wet out with resin immediately prior to placing the fabric in the hull mold, or the resin is applied directly to fabric which has been draped inside the mold. Resin is forced through the fabric with hand rollers and squeegees to ensure saturation of the reinforcing fiber. Skilled labor is required to reduce the amount of air trapped in the laminate, and the ratio of resin to fiber (which largely determines the weight and mechanical properties of the finished laminate) is difficult to control.
Closed-mold processes using resin infusion under vacuum can dramatically improve the mechanical properties of conventional laminates, but they require a complex mold whose cost can only be justified if a large number of components are to be produced from the mold.
Pre-preg resin systems have also greatly improved the laminating process. Oversimplified, a carefully measured amount of pre-mixed resin and hardener is injected into the reinforcing fiber material, which is drawn through a series of rollers to thoroughly squeeze the resin throughout the fabric. The ratio of resin to fiber can be controlled in a way that is virtually impossible with conventional wet layup. The goal is for the finished laminate to contain just enough resin to hold the reinforcing fibers in position in the laminate. Resin-rich laminate is a no-no in state-of-the-art construction. Epoxy resins can be modified so that the cross-linking that converts the material from a liquid to a solid is only completed when the resin/hardener mixture is raised to a certain temperature. The types of epoxy resins used by most amateurs for repair and construction cure around room temperature, so that once the resin and hardener are mixed, it becomes a battle against time to complete the layup before the resin sets.
The epoxy resins used for high-tech construction are formulated to cure when their temperature is raised to a specific level, typically about 95 degrees Celsius, or 200 degrees Fahrenheit. This may seem like an arbitrary temperature, but it is not. Even in state-of-the-art construction, male plugs are typically made of wood, which is then covered with polyester or epoxy fairing materials to smooth and seal the surface. Even the driest wood contains water. If the wooden plug is raised above 100 Celsius (212 Fahrenheit) while the laminated hull is curing, residual moisture in the wooden plug will evaporate, and the shape of the plug will change, distorting the final hull shape.
Heat is required to complete the cross-linking process which converts the resin to a solid. The pre-impregnated fabric is stored in huge freezers until 24 hours or so before it is scheduled to be used, at which time it is slowly brought up to room temperature. At room temperature, the resin becomes tacky, allowing the fabric to be carefully layered in the mold or on the plug. The tackiness of the resin holds the fabric in place as layer on layer is put down. The fiber orientation of the reinforcing material is pre-determined by the laminate engineer to insure that the finished part has the proper mechanical properties. It is up to the laminators to follow the laminate schedule drawings to the letter. There is no unskilled labor in the building of high-tech composite components.
Core Materials
Virtually every modern composite boat is of cored construction. The production boatbuilding industry has been largely limited to balsa and various foams for core materials. Balsa and foam cores are relatively cost-effective and easy to handle, since they do not absorb significant amounts of resin from the laminate while it is curing, and have good mechanical properties when used in sandwich construction.
For state-of-the-art construction, however, honeycomb cell cores of aluminum or Nomex are commonly used. The interface between the fiber laminate and the core material is the most critical part of cored construction. This bond is make-or-break. If there is not enough “glue” in this joint, the core and skin can separate, particularly under impact. If there is too much adhesive, the full mechanical properties of the sandwich may not be achieved, and the part will be heavier than designed.
In typical male plug construction, the inner skin, the film adhesive that bonds the core to the skin, and the core itself, are laid up together, placed under a vacuum, and cooked at one time. The outer skin is then laid up over the cured inner skin and core, once again using a film adhesive. Before cooking, the skins are “de-bulked”—compacted under vacuum at a temperature well below cure temperature—to remove air from the laminate and squeeze the layers of fiber and resin tightly together.
A few brave souls have cooked inner skin, outer skin, and core in one shot, but the difficulty of evacuating all the air from the laminate during the process makes it a risky proposition with a serious downside. You could easily end up with a gigantic carbon fiber soufflé with dramatically compromised structural properties.
Engineering
For a racing sailboat, designers and builders will go to unbelievable lengths—and sometimes staggering costs—to make the hull and deck as light as possible. For a given displacement, the lighter the hull, deck, hardware, and rig, the more weight can go into the ballast keel.
At the extreme are the boats of the America’s Cup Class. From 1992 until 2003, the construction requirements of the class were largely unchanged. Maximum displacement was limited to 25 metric tons, or about 55,000 pounds. Due to quirks in the rating formula, it was advantageous to built to the maximum weight limit.
The minimum weight of the rig is fixed. There are minimum core density and skin weight requirements, but engineering a structure capable of carrying the loads of the rig and keel is up to the individual designer. There is no plan review by a classification society to make sure the structure meets any minimum standard. It was quickly determined that a long, narrow boat, with as much of the all-up weight as possible jammed into a ballast bulb 13 feet below the surface, was the quickest way to get upwind. Getting to the weather mark first means winning the race 70 percent of the time.
With the minimum weight of the hull shell largely controlled by the class rule, but the weight of internal supporting structure essentially ungoverned, it became a battle to get weight out of the structure. The answer was a dramatic refinement of hull shell engineering and construction, to the point where much of the internal reinforcement was incorporated in the hull shell itself, rather than into framing, stringers, bulkheads, or other support structures. This is best seen in the change in the percentage of the total weight of the boat incorporated in the ballast keel over a 10-year period. In 1992, a typical ballast bulb might constitute 38,500 pounds of the 55,000 pound weight of the boat, for a ballast/displacement ratio of 70 percent. This is high, but not extraordinary.
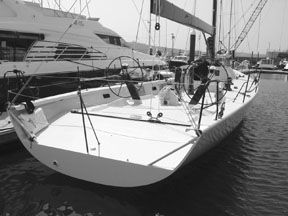
By 2003, however, you found over 45,000 pounds in the bulb, yielding a ballast/displacement ratio of more than 80 percent. This may be the highest ballast/displacement ratio ever in a “conventional” ballasted monohull.
While some of this weight loss was the result of lighter keel fins, booms, spinnaker poles, hardware, instrument systems, and running rigging, a good chunk of it came from improved structural engineering of the hulls and more sophisticated boatbuilding techniques. Of course, there have been more than a few structural failures along the way—some of them quite spectacular. Life at the cutting edge is not cheap, or risk free.
Conclusions
It’s not just the America’s Cup that leads to improvements in engineering and construction. The Volvo 70 ocean racers, with their can’ting keels and incredible power-to-weight ratios, will bring design, engineering, and construction of offshore sailboats to a new level as these boats are built over the next year. Among slightly more conventional racing boats, the Transpac 52s employ virtually identical engineering and construction methods. The next time you look at one of these racing thoroughbreds, either in the flesh or in photographs, look carefully at the details. While much is proprietary and hidden from view, much is right in front of you, if you’ll take the time to look closely.
No, most of this stuff is not going to show up on the new 40-foot cruising boat you buy next year, or even five years from now. But just as racing improves the breed when it comes to horses, the same goes for boats. In shops around the world, designers and builders are working on the cutting edge. Faster, stronger, and lighter is their credo. From their successes—and from their failures as well—come many of the developments that you will see in new boats.